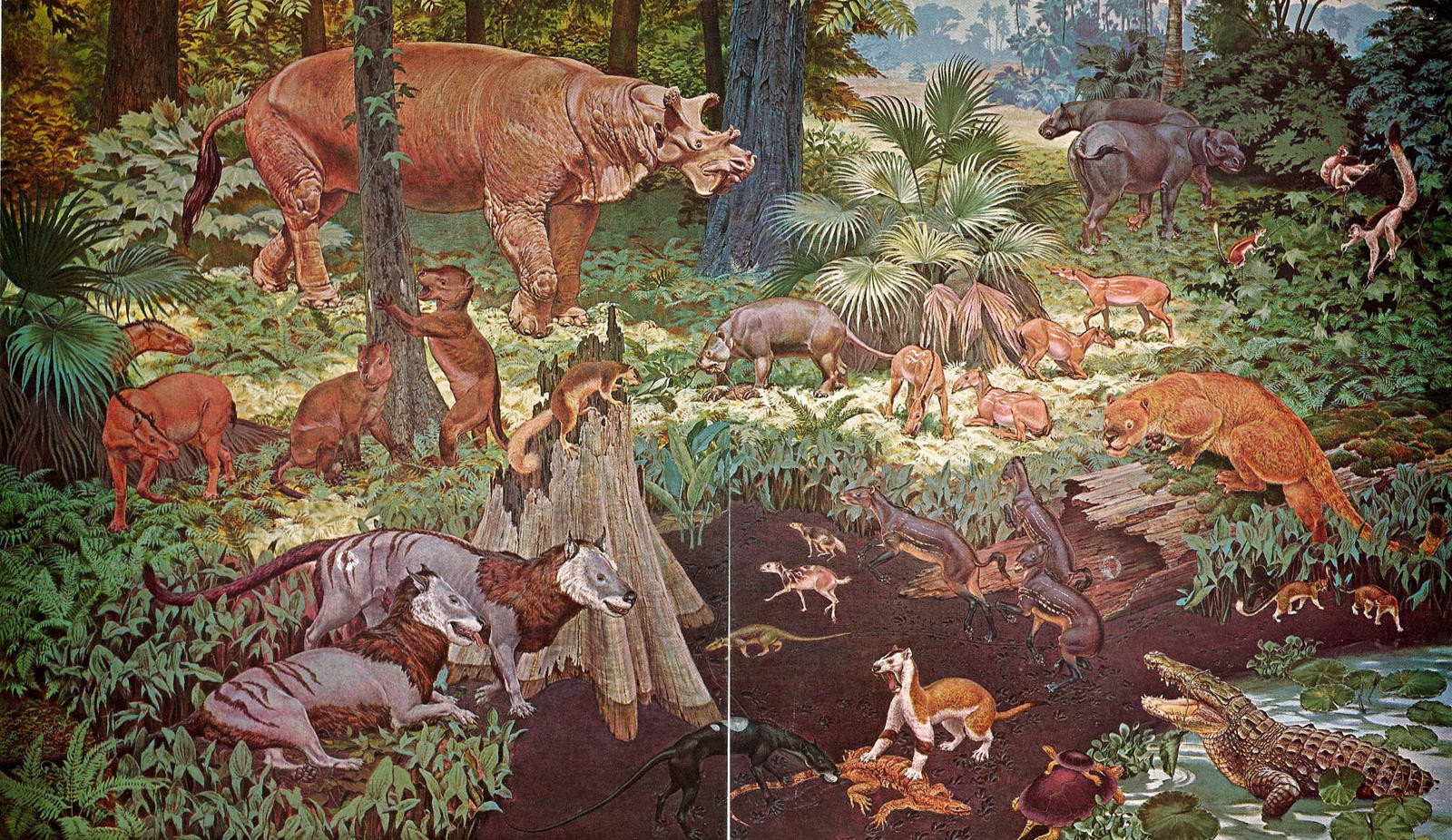
Tomorrow's forecast
Tiny fossils yield big clues on climate change
The vast expanses of deep freeze we see today haven’t always covered the Earth’s polar regions. For much of our planet’s past, the poles sported no caps of snow and ice. In fact, about 56 million years ago, one could describe polar conditions as downright balmy, with alligators, turtles, creodonts, and tapirs frolicking amidst towering palm trees and humid, lush swamps.
Thanks for creating this polar idyll goes to a massive release of carbon into the atmosphere over roughly 5,000 years. This big exhale caused the Earth at the time to spike a 150,000-year-long fever, with temperatures rising more than 5° C. Scientists think an enormous wave of volcanic eruptions triggered this warming event, known as the Paleocene Eocene Thermal Maximum, or PETM. The heightened volcanic activity occurred as the Earth’s crust fragmented at the transition between the Paleocene and Eocene epochs when the continental land masses were moving to their current positions. Floods of magma combusted organic matter buried in sediments, releasing a surge of CO2. The resulting warming of a few degrees started a positive feedback loop, jacking up ocean temperatures to drive further releases of greenhouse gases, and further warming.
How do we know this? Much of it from the work of distinguished professor of Earth and planetary sciences and Ida Benson Lynn professor of ocean health James Zachos, one of the first scientists to delineate PETM-related climate changes and what caused them. Using advanced analytical chemistry and marine geology tools, Zachos’s research looks back in time to reconstruct the details of how the Earth’s climate fluctuated in the distant past. In addition to improving our understanding of the links between global temperature, ocean acidification, and the carbon cycle, the research importantly hints at the future challenges we might face from the potential fallout of our current climate crisis.
Although the 5,000 years that began the PETM sounds like a long time, it’s a mere blink of an eye on a geological scale. Today, the Earth’s climate is changing perhaps 10 or even 100 times faster than it did then, as our burning of fossil fuels and other activities continue to dump roughly 5.5 petagrams (1 petagram = 1 billion tonnes) of carbon into the atmosphere each year. “We know now that even if we were to shut off carbon emissions today, the effects will last for thousands of years,” Zachos said. “We are leaving a legacy for many future generations of humans.”
Sudden shake-ups
In the late 1980s when Zachos was a graduate student, scientists had just begun exploring the idea of using the ratios of different isotopes of carbon and other elements in fossils to detect and characterize past geological events. For his doctoral dissertation, Zachos applied the idea to zero in on the end of the Cretaceous period, 66 million years ago, when an extinction event—most think a meteorite impact—wiped out the dinosaurs and many other terrestrial and marine organisms. The work involved tracking the ratio of carbon 12 to carbon 13 present in the fossilized shells of tiny free-floating ocean organisms called microplankton. Essentially, the fossilized shells record the chemistry of the seawater their makers lived in. Having settled on the ocean floor, they create an archive in the sediment.
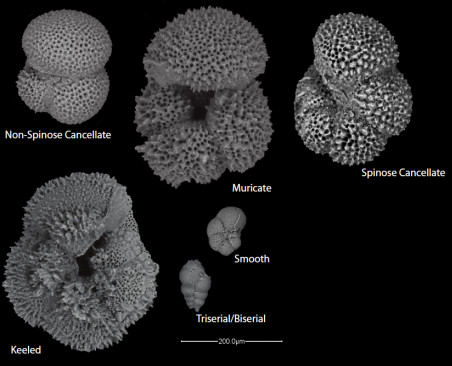
Reading his shell fossil samples from the end of the Cretaceous, Zachos found that the ratio went haywire. Biologically sustained patterns in carbon isotope ratios suddenly disappeared, suggesting a major breakdown in carbon fixation in the ocean. “That started me thinking about the connection between environmental perturbations and the carbon cycle,” he said. As Zachos recollects, he noted at the time that most boundary transitions between geological epochs were associated with sudden shake-ups in animal and plant life. And unlike the meteorite-doomed Cretaceous, most other transitions weren’t associated with a clear event. Might these boundaries reflect dramatic changes in climate, he wondered? And might that change be encoded in the geological record?
In the PETM, while many marine and other organisms went extinct, many of the mammals, including primates, that still exist today first appeared on the planet. The period has provided a fascinating test case that Zachos continues to study to this day. Initially, though, he teamed up with distinguished professor of Earth and planetary sciences and dean of physical and biological sciences Paul Koch, then a postdoc at the Smithsonian National Museum of Natural History, to probe the carbon isotope ratio shifts in mammalian fossils from the period. At first, they couldn’t quite put it together, Koch said. “Jim actually called me and said, ‘Hey, the numbers look really weird.’”
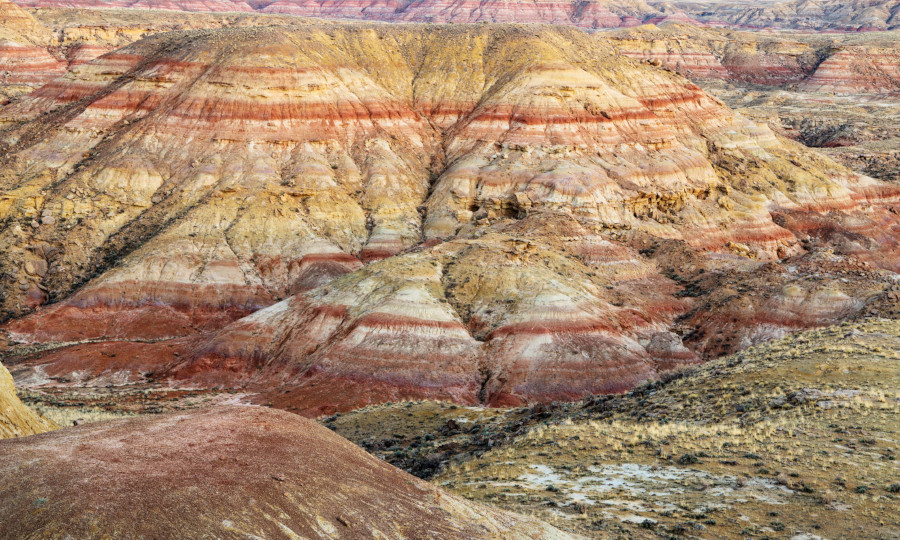
But then, Koch said, a breakthrough study was published that used a similar carbon analysis on a sediment core from the ocean near Antarctica to link—for the first time—a temperature jump during the PETM of as much as 7° C to a major perturbation in the carbon cycle. “They identified this event in the ocean, and we quickly realized that we had found the same event on land,” he said. “It was crystal clear.”
Six months later, in 1992, Koch, Zachos, and colleague and now professor emeritus at the University of Michigan Philip Gingerich published their own paper in the prestigious journal Nature. It was the first to connect a major global warming event with large-scale carbon cycle fluctuation, speciation, and mass extinction. “It was like two pieces of a puzzle snapping together,” said Scott Wing, research geologist and curator of paleobotany at the Smithsonian National Museum of Natural History, who began an ongoing collaboration with Zachos shortly afterwards.
Finding connections
That study provided an entry point into understanding how warming events can affect different aspects of the Earth’s biosphere. The first step involved seeing whether some of the carbon that drove the surge in temperature was absorbed by the ocean, as evidenced by an increase in its acidity. To find out, Zachos submitted a proposal to conduct a study with the Ocean Drilling Program, a multinational venture aimed at establishing a basic understanding of ocean basins and the Earth’s crust underlying them. Zachos and his colleagues set out to sample sediment cores from deep in the ocean floor at multiple spots across the Atlantic and the Pacific in order to try and detect a change in the ocean’s carbon chemistry. Within a decade, the effort had gathered enough evidence to document, in the brief period of the PETM’s first 5,000 or so years, dramatic ocean acidification, evidence of the release of as much as 5,000 petagrams of carbon into the atmosphere. “On a geologic time scale, a petagram of carbon per year is a lot,” Zachos said. “It’s enough that the system can’t buffer itself.”
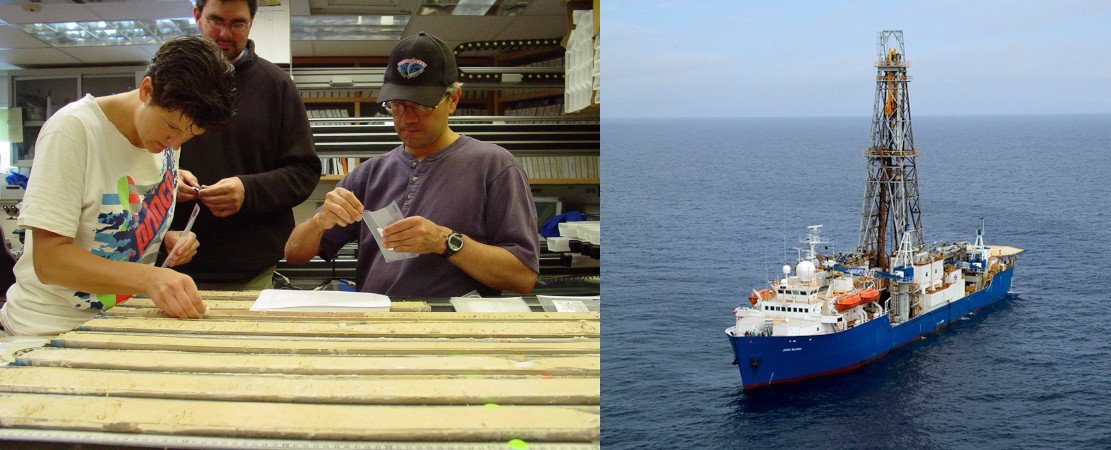
Since then, Zachos and his team have worked to improve their measurements, which has allowed them to quantify a variety of climate parameters at finer time intervals and with increased spatial precision. For example, Zachos collaborated with Bärbel Hönisch, professor of Earth and environmental sciences at Columbia University, to more accurately measure ocean acidification in different places and times in the geological record. Using mass spectrometry, they measured the ratio of boron-11 to boron-10 in the fossilized shells of foraminifera, a popcorn-shaped type of microplankton. At higher pH, the ratio increases, such that it provides a quantifiable measure of ocean pH, Hönisch said.
Such increasingly sophisticated techniques have allowed Zachos and his team to ramp up the speed and types of measurements they can make. Meanwhile, computer models created to predict current climate trajectories have also grown more sophisticated, able to account for potentially confounding climate variables such as cloud cover and ocean circulation. Of course, conditions 56 million years ago are not the same as today, so the effects of rising atmospheric carbon won’t match up exactly. But by comparing their increasingly precise real-world—but old-world—data from the PETM and other warming events in geological history with the results of today’s computer modeling, Zachos and his colleagues are anchoring future climate predictions in the context of a past reality. In a way, said Wing, understanding the fine details of how climate change played out in the past allows researchers to test how well current models of future climate change actually work. “If you are interested in what’s going to happen in the next 1000 years, you need to be able to describe the past at a similar resolution,” he said.
The forecast?
What the data show is a rate of carbon emission about an order of magnitude higher today than during the PETM—which translates to us taking a few hundred years versus a few thousand to belch the same amount of carbon into the atmosphere. How might this affect us? “We are recognizing that the rate of global warming and sea-level rise will be faster than we had predicted in the past,” said Zachos.
The data also provide an answer to the question of how long it might take for the planet to recover. During the PETM, naturally occurring chemical processes such as rock weathering—in which rain, which is slightly acidic, gradually breaks down rock to form calcium bicarbonate that then gets stored in the ocean—eventually returned the carbon cycle to a more even keel. “What we found is that it took tens of thousands of years for the excess carbon to be removed through such natural processes,” Zachos said.
The specific mechanisms—the roles played, for example, by changes in vegetation, or carbon absorption in the deep ocean—that drove the return to baseline during the PETM and other more recent climate cycle fluctuations remain an active area of investigation for Zachos and his collaborators. Another major focus is how greenhouse-induced changes in the hydrologic cycle—the transfer of water from the surface to the atmosphere and back again—might drive changes in precipitation, such as more frequent and intense storms. Many aspects of the climate physics involved in this question remain unclear. But unraveling how factors like energy, ice, and water moving between the different layers of the atmosphere shaped the dynamics of past climates could provide important insights on what our future might hold.
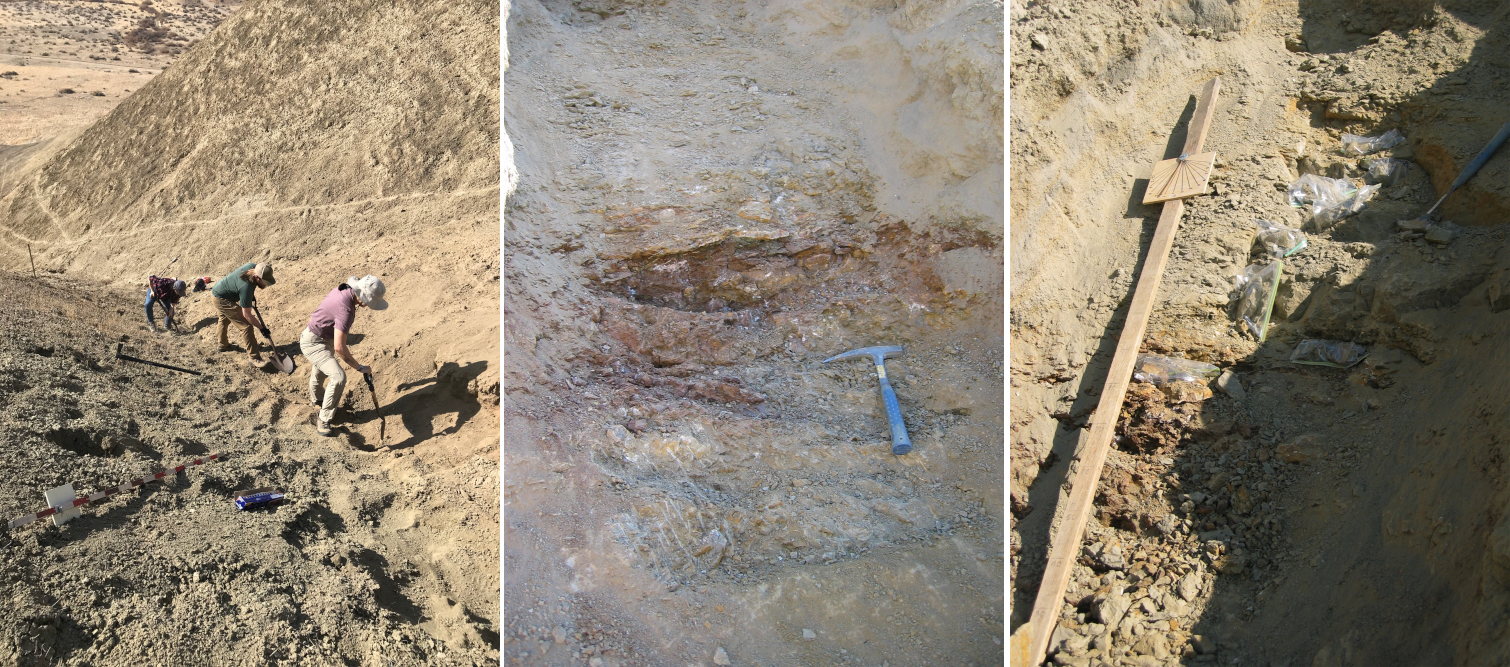
While the PETM saw a surge in the diversity of mammals, including our first primate ancestors, researchers have surmised that—even for creatures cavorting in the then subtropical polar climes—life was difficult. Extinctions aside, fossils from the period reveal abnormal dwarfing in animals and plants with reduced levels of nutrients. And the sedimentary studies of Zachos and others indicate it was a period marked by an enormously variable climate, with intense precipitation, flooding, and landslides. In answer to his own question, “Is that what our future will look like, but exaggerated?” Zachos said, “Yeah, I think the evidence from the past suggests that’s exactly what’s going to happen.”
The toll will be heaviest on the most vulnerable, said Hönisch, on those—humans and other living creatures alike—without the means to move to cooler or higher regions as the planet heats up and the oceans rise. But just as it did 56 million years ago, the planet will make it through, Hönisch said. “The planet will help itself,” she said, “and I hope we will survive as a species, too.”